ACROSS THE LIFESPAN
Stress
individual variation in responding to mild to moderate stressors:
-
person’s perception
-
sense of control
-
regulation of immune function
Thus perception also influences resistance and susceptibility to disease
Stress also can contribute to sleep loss when people get caught in a vicious cycle: elevated glucocorticoids delaying the onset of sleep, and sleep deprivation raising glucocorticoid levels.
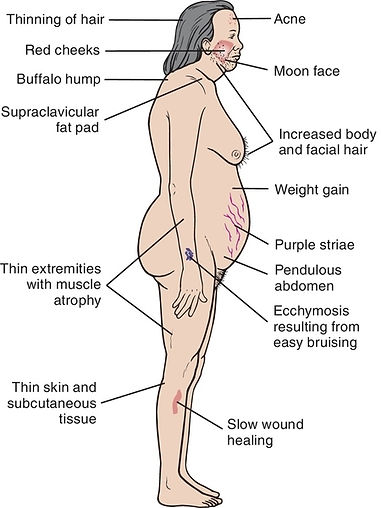
What is Stress?
-
fundamental process
-
psychological, physical, or both
-
external stimulus that threatens homeostasis
-
affects all organisms, from the simplest bacterium and protozoan, to complex eukaryotes such as mammals
-
can be induced by the belief that homeostasis might soon be disrupted
-
response to impending danger
-
muscles are primed
-
attention is focused
-
nerves readied for action
-
Lack or loss of control is a particularly important feature of severe psychological stress, which can have physiological consequences.
Acute stress is good BC:
-
improve memory
-
boost immune function
-
enhance muscular activity
-
restore/ maintain homeostasis
-
provide the extra strength and energy needed to cope
-
protect the body from immediate danger (ex. pathogens)
worst stress is chronic:
-
colitis
-
diabetes
-
hypertension
-
atherosclerosis
-
abdominal obesity
-
irregular menstrual cycles in females
-
impotency and loss of sex drive in males
-
can upset the body’s biochemical balance and accelerate disease
-
repeated elevation physiological stress responses or fail to shut them off
molecules have evolved to provide a series of emergency systems that protect key cellular functions from unexpected external challenges and their internal consequences.
Ex. special molecules called heat-shock proteins guide damaged proteins to where they can be repaired or harmlessly degraded, thus protecting cells from toxicity or dysfunction.
A stressful situation activates three major communication systems in the brain, all of which regulate bodily functions
first found these from experiments, primarily with rats, mice, and nonhuman primates such as monkeys
-
VNS (voluntary nervous system), which sends messages to muscles so that we may respond to sensory information.
-
Ex., the sight of a shark in the water may prompt people to run from the beach as quickly as possible.
-
-
ANS, made up of the symp and the parasymp branches. Each has a specific task in responding to stress.
-
SNS
-
arteries supplying blood to the muscles to relax in order to deliver more blood, allowing greater capacity to act.
-
blood flow to the skin, kidneys, and digestive tract is reduced.
-
Npe is released by nerves; Epe is released by the adrenal glands
-
They activate R`s in BV and other structures, these substances ready the heart and working muscles for action.
-
-
PsNS
-
regulate bodily functions and soothe the body once the stressor has passed, preventing the body from remaining in a state of mobilization too long.
-
If these functions are left mobilized and unchecked, disease can develop.
-
calming actions: reduce the harmful effects of the emergency branch’s response to stress.
-
ACh released, producing calming effects.
-
-
-
neuroendocrine system, which also maintains the body’s internal functioning.
-
also maintains the body’s internal functioning
-
Various stress hormones travel through the blood and stimulate the release of other hormones, which affect bodily processes such as metabolic rate and sexual function.
-
Hormones underpin the fight or flight response - the classical, immediate reaction that has to be made in response to danger.
Symtoms:
initial tingling sensation
sweating
heightened awareness
rapid pulse rate
higher blood pressure
general feelings of fear
Neuroendocrinology: brain - hormones, nutrients, inflammatory molecules, and with information from peripheral nerves monitoring vital organs and sensations
Glucocorticoids and epinephrine =both synthesized in the adrenal glands, signal to mobilize glucose into the blood.
The hypothalamic-pituitary-adrenal (HPA) axis
Major responses to stress
-
Immediate reaction; tingling sensation, sweating, heightened awareness, rapid pulse rate, higher blood pressure and general fear
-
receptors on blood vessels, causing BV constriction and BP to shoot up, heart accelerate and produce the pounding sensation in the chest known as palpitations
-
receptors in skin causing hairs to erect (goosebumps) and in the gut causing those disconcerting abdominal sensations that we all sense as stress
-
-
neuroendocrine response; activate HPA axis.
-
hypothalamus, pituitary gland, adrenal cortex and hippocampus by a bloodstream highway carrying specialised hormones
-
Hypothalamus regulates hormones.
-
inputs from
-
areas processing emotional information
-
amygdala
-
regions of the brainstem controlling SNS responses
-
-
It integrates these to produce an output
-
Corticotrophin releasing hormone (CRh) is released from the hypothalamus and travels to the pituitary gland
In the Pituitary Gland
-
CRh triggers the release of adrenocorticotropic hormone (ACTh)
-
ACTh travels in the blood to the adrenal glands, where it stimulates the release of cortisol
-
releases ACTH into the blood.
-
ACTH then stimulates a part of the adrenal gland to secrete cortisol.
-
The physiological changes induced by the costisol after it has been secreted by the adrenal glands affects the body just as importantly as it affects the brain;
-
hippocampus, a key structure for learning and memory, but cortisol also acts on the
-
amygdala, which processes fear and anxiety
Glucocorticoids
Cortisol is a steroid hormone
Supervised by the Hus, the AGs secrete glucocorticoids
This produced an array of effects in response to stress
Some of the actions of glucocorticoids help mediate the stress response, while other, slower actions counteract the primary response to stress and help re-establish homeostasis.
-
raises blood sugar and other metabolic fuels such as fatty acids
-
helps adrenaline to raise blood pressure and, in the short term, makes you feel good
-
turns off growth, appetite and digestion, inflammation, woundhealing, sex
-
often occurs at the expense of proteins that are broken down into fuels required immediately - instant ‘chocolate bars’ for the muscles and brain
The last step of the circuit is cortisol feedback to the brain.
The highest density of cortisol receptors in
-
hippocampus, a key structure for learning and memory, but cortisol also acts on the
-
amygdala, which processes fear and anxiety
The net effect is to turn on the amygdala- to allow learning of fear-related information; and to turn off the hippocampus - to ensure that resources are not wasted on more complex but unnecessary aspects of learning. Cortisol is focus juice.
Over the short run, epinephrine mobilizes energy and delivers it to muscles for the body’s response.
The glucocorticoid cortisol promotes energy replenishment and efficient cardiovascular function.
Glucocorticoids also affect food intake during the sleep-wake cycle.
Cortisol levels, which vary naturally over a 24-hour period, peak in the body in the early-morning hours just before waking.
-
helps produce a wake-up signal
-
turning on:
-
appetite
-
physical activity
-
This effect of glucocorticoids may help explain disorders such as jet lag, which results when the light-dark cycle is altered by travel over long distances, causing the body’s biological clock to reset itself more slowly. Until that clock is reset, cortisol secretion and hunger, as well as sleepiness and wakefulness, occur at inappropriate times of day in the new location.
Cortisol and epinephrine facilitate the movement of immune cells from the bloodstream and storage organs, such as the spleen, into tissue where they are needed to defend against infection.
Glucocorticoids do more than help the body respond to stress; help the body respond to environmental change. In these two roles, glucocorticoids are in fact essential for survival.
2 important roles of Glucocorticoids:
help the body respond to
-
stress
-
environmental change
Chronic Stress
release of hormones (glucocorticoids and epinephrine) to improve memory, boost immune function, enhance muscular activity, and restore physiological balance overuse can lead to consequences which can be negative: memory is impaired, immune function is suppressed, and energy is stored as fat.
Overexposure to glucocorticoids leads to weakened muscles.
Elevated glucocorticoids and epinephrine contribute to:
-
hypertension
-
atherosclerosis
-
abdominal obesity
-
memory is impaired
-
energy is stored as fat
-
immune function is suppressed
-
sleep loss (SD cause inc. GCTD)
Epinephrine also increases the release and activity of body chemicals that cause inflammation, adding to the body’s chronic stress burden. This continual chemical activity can lead to arthritis and accelerated aging of the brain.
Aging rats show impaired neuron function in the hippocampus — an area of the brain important for learning, memory, and emotion — as a result of increased glucocorticoid secretion throughout their lives.
Overexposure to glucocorticoids also increases the number of neurons damaged by stroke.
prolonged glucocorticoid exposure before or immediately after birth causes a decrease in the normal number of brain neurons and smaller brain size.
chronic stress:
-
colitis
-
diabetes
-
hypertension
-
atherosclerosis
-
abdominal obesity
-
irregular menstrual cycles in females
-
impotency and loss of sex drive in males
Stress and The Brain
The hippocampus has high levels of the two receptors for cortisol
-
low MR Mineralocorticoid Receptor
-
high GR Glucocorticoid receptors
The low MR receptor is activated by the normally circulating levels of cortisol in the bloodstream highway of the HPA axis. This keeps our general metabolism and brain processing ticking over nicely. However, as cortisol levels begin to rise, particularly in the morning, the high GR receptor becomes progressively more occupied.
When we become stressed, cortisol levels become very high indeed, activation of this receptor is sustained and the hippocampus is then shut down by a genetically controlled program. Put all this together and you have what is called a bell-shaped curve. This is the classical curve relating stress to brain function - a little bit is good for you, a bit more is better, but too much is bad!
The immune system receives messages from the nervous system, and it is also sensitive to many of the body’s circulating hormones, including stress hormones.
short-term elevations of stress hormones = immune function and protective against disease pathogens
long-term glucocorticoids = suppress immune system, w/ negative consequences
however, glucocorticoid-induced immunosuppression also has its benefits.
Normally, glucocorticoids rein in the immune system boost brought on by stress. Without this brake, there is an increased chance of autoimmune disorders caused by an overactive immune system.
autoimmune disorders occur when the body’s immune defenses turn against itself
individuals must be treated with synthetic forms of glucocorticoids (e.g., hydrocortisone and prednisone) in order to keep the immune system in check.
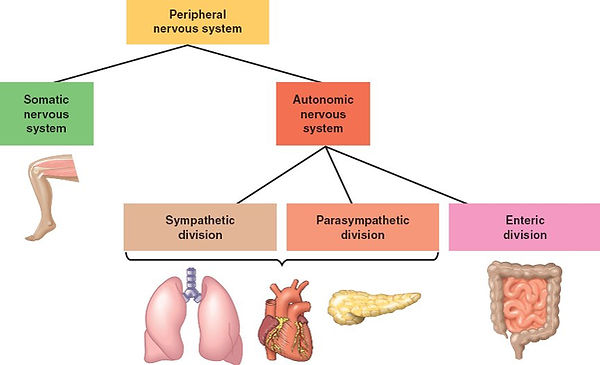
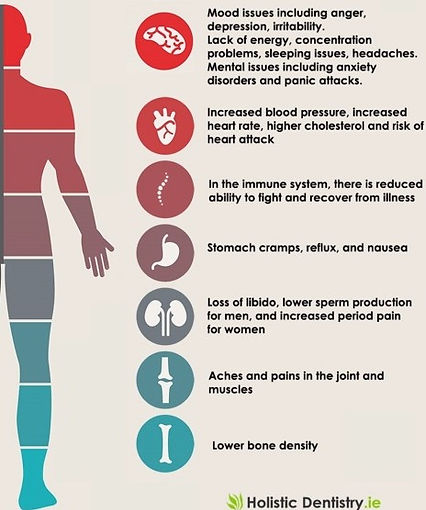
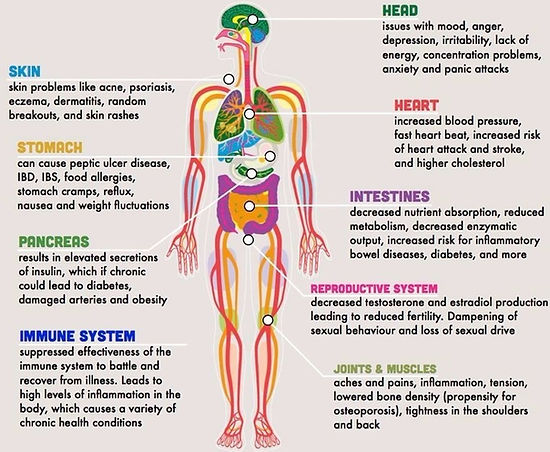
People at greatest risk of stresses effects:
-
high demand low control Jobs
-
ex. telephone operators, waiters, and cashiers
higher rates of heart disease than people who can dictate the pace and style of their working lives.
-
hostile and irritated by trivialities
-
high hostility
-
larger increases in levels of stress hormones
-
muscle blood flow
-
blood pressure
-
-
learning to reduce or avoid anger could be important to avoid cardiovascular damage.
Caused by:
-
receptors on blood vessels to constrict
-
BP and HR accelerate (palpitations)
-
-
receptors in the skin and gut
-
hairs to erect
-
gut; abdominal sensations
-
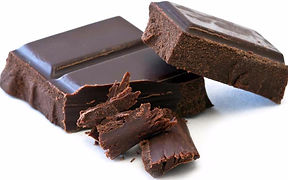
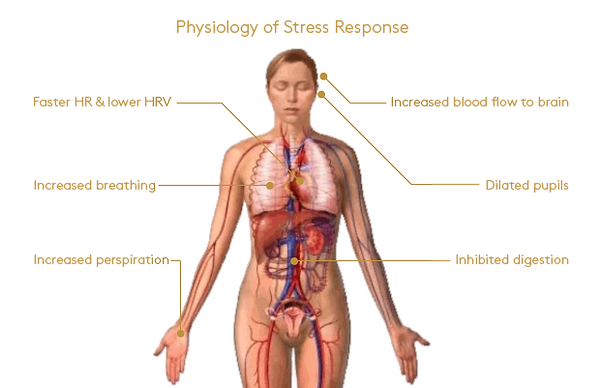


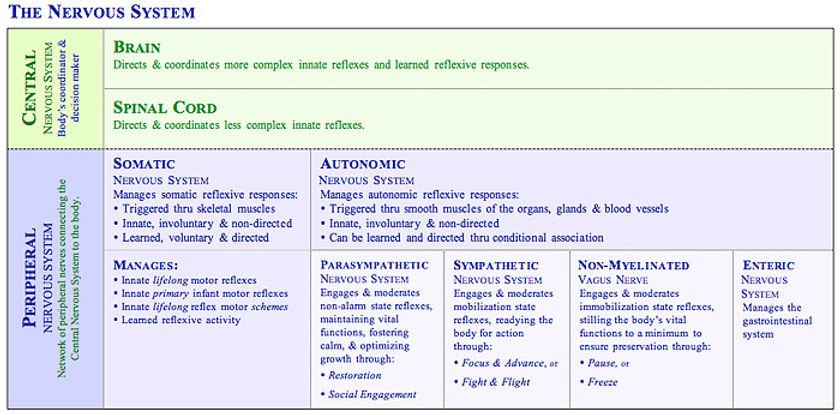
tHE IMMUNE SYSTEM
Until just a few years ago, the brain was thought to be an “immune privileged organ” because it was not affected by immune responses or inflammation.
“blood brain barrier”. This is not really a barrier, but specialised endothelial cells in the brain blood vessels that are relatively resistant to the passage of large molecules or immune cells from the blood into the brain.
Neuroimmunology is now a very active area of research.
The immune system is our first line of defence against malicious invaders:
viruses, bacteria, yeast
range from common and mild, such as the all too familiar cold, to severe and life threatening, e.g. HIV, meningitis or tuberculosis.
How does the Immune System react to harm?
-
locally; within the tissue that is infected, injured or inflamed, causing swelling, pain,
-
changes in blood flow and release of local inflammatory molecules
-
activation causes:
-
leucocytes and macrophages and acute phase proteins that travel to the site of attack, to identify, kill and then remove invading pathogens.
-
In addition, the acute phase response generates the symptoms we have all felt (fever, aches and pains, sleepiness, loss of appetite, disinterest).
-
-
-
Each of these responses helps to combat infection, conserve energy and aid repair, but when activated too much or for too long they can be very damaging. Careful control must be imposed.
Immune Signaling Systems
The brain receives signals from injured or infected tissues that may be neural in origin (via sensory nerves) or humoral (via circulating molecules).
Neural signals seem to be via Cfibres and via the vagus nerve from the liver – a key site for production of acute phase proteins.
The nature of the main circulating signals to the brain are not fully understood, but are believed to include prostaglandins (which are inhibited by aspirin), and complement proteins (a cascade of proteins important in killing invader cells).
But perhaps the most important signals are a group of proteins which came to light only in the last 20 years – known as cytokines.
Cytokines are the body’s retaliators. There are now well over 100 of them - and more are being discovered all the time. These proteins are normally produced in the body at very low levels, but are switched on quickly in response to disease or injury.
They include interferons, interleukins, tumour necrosis factors and chemokines. Many are produced locally within damaged tissues and act on cells nearby, but some enter the blood stream where they send signals to distant organs including the brain. It is cytokines that cause most of the responses to disease and infection.
The triggers for cytokine production
bacterial or viral products
damage to cells
threats to cell survival (ex. toxins or low levels of oxygen)
Another important regulator of cytokine production is the brain that, through neural signals to tissues (mainly via SNS) or hormones (such as cortisol from the adrenal gland), can switch cytokines on or off.
Cytokines have many actions, esp. on the immune system.
-
Most stimulate the immune system and the key components of inflammation such as swelling, local changes in blood flow, and the release of a second wave of inflammatory molecules.
-
They act on almost all physiological systems, including the liver where they stimulate the acute phase proteins.
-
However, although cytokines share many actions, they also vary significantly.
-
Some are anti-inflammatory and inhibit pro-inflammatory process; most act locally on cells close to where they are produced, while others are released into the circulation, like hormones.
-
Stress affects HPA axis and immune system, via BRAIN
-
production of a protein in the hypothalamus called CRF.
-
CRF travels the short distance from the hypothalamus to the pituitary gland to release another hormone, ACTH.
-
ACTH travels via circulation to the adrenal gland to release steroid hormones (cortisol in humans)
-
cortisol of the most potent suppressors of immune function and inflammation
-
But the story seems to be more complex than this because there are other hormonal and neural elements, and we also know that some forms of mild stress can actively improve our immune function
-
Recent research has shown that many of the defence molecules such as cytokines are also active contributors to brain diseases such as multiple sclerosis, stroke and Alzheimer’s. It seems that overproduction of defence molecules within the brain itself can damage neurons - particularly certain cytokines.
Various new treatment strategies for brain disease are now being developed with the idea of inhibiting immune and inflammatory molecules.
AGING
In 1900, the ALE was about 47 years. At that time, three million people, or 4 percent of the population, were older than age 65, and they were typically in ill health.
By 2007, ALE reached approximately 78 years, and today, more than 39 million people, or almost 13 percent of the population, are older than age 65.
That said, however, almost everyone gets a bit forgetful in old age, particularly in forming memories of recent events.
For example, once most people reach their 70s, they may find themselves forgetting names, phone numbers, and where the car is parked more frequently. In addition, people might respond more slowly to conflicting information.
These behaviors are not signs of disease.
they are considered part of the normal aging process.
There are, however, a small number of individuals whose mental functioning seems relatively unaffected by age. In fact, the wisdom and experience of older people often make up for deficits in performance. The oldest known human, Jeanne Calment, kept her wits throughout her 122-year life span. Unfortunately, some individuals do develop dementia, a progressive and severe impairment in mental function that interferes with the activities of daily living.
The term dementia includes a number of different diseases, of which Alzheimer’s disease is the most common.
Other dementias include cerebrovascular disease, Pick’s disease, and Lewy body disease. Together, the dementias affect as many as 6.8 million people in the U.S, and at least 1.8 million of those cases are severe.
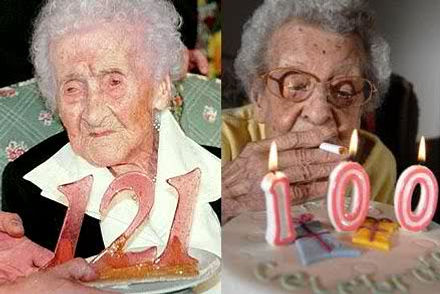
We now know that the brain reaches its maximum weight near age 20, and subtle changes in the brain’s chemistry and structure begin at midlife for most people.
During a lifetime, the brain is at risk for losing some of its neurons, but normal aging does not result in widespread neuron loss. This distinguishes normal aging from the neurodegenerative changes that occurs as part of the disease process in Alzheimer’s or Parkinson’s disease or after a stroke.
Brain tissue can respond to damage or loss of neurons in several ways. The remaining healthy neurons are able to expand their dendrites and fine-tune their connections with other neurons. If the cell body of the neuron remains intact, a damaged brain neuron can readjust by inducing changes in its axon and dendrites.
Thus, damage/loss = Healthy neurons reaching out and Fine tune their connections
Relatively small stem cell populations remain in a healthy adult brain, but our current knowledge suggests that they contribute to only a few of the many different types of neurons found, and these neuron types are found in only a few regions of the normal brain. Compounding the problem is the fact that the number of even these stem cells declines as part of the aging process.
Longitudinal Study Results show
decline in some mental functions and improvements in others.
-
speed of carrying out certain tasks becomes slower, but vocabulary improves.
-
less severe declines in the type of intelligence relying on learned or stored information (crystallized) compared with the type that depends on the ability to deal with new information (fluid).
This research is supported by animal studies in which scientists find that changes in mental function are subtle.
-
Ex. rodents and primates with only minor detectable brain abnormalities, certain spatial tasks, such as navigating to find food, tend to become more difficult with age.
-
Studies show that many areas of the brain, especially in the cortex, maintain most of their neurons throughout life. The connectivity between neurons changes with aging, indicating that the brain is capable of being modified or improved.
-
It is also becoming clear that the aging brain is only as resilient as its circuitry. Scientists debate whether this circuitry is changed by neuron atrophy alone or whether some neuron loss over time is inevitable. In any event, when the circuitry begins to break down, remaining neurons can adapt by expanding their roles, and larger portions of the brain can be recruited so that older people can reach performance levels similar to those of younger adults.
Thus, the more resilient the brain, the more likely for remaining neurons to expand their roles to accomodate for neuronal atrophy to reach preformance levels of youngsters.
In addition, learning conditions may dictate what happens to brain cells. Studies of rats shed light on some of the changes that occur in brain cells when the animals live in challenging, stimulating environments. Middle-aged rats exposed to such environments formed more and longer dendrite branches in the cerebral cortex than did rats housed in isolated conditions.
In response to enriched environments, older rats tend to form new dendrite outgrowths and synapses, just as younger animals do. But the response is more sluggish and not as large. Compared with younger rats, older rats have
less growth of the new blood vessels that nourish neurons.
Another study showed that when rats were given acrobatic training, their brain cells had more synapses per cell than rats given only physical exercise or rats that were inactive. These findings led scientists to conclude that motor learning generates new synapses. Physical exercise alone, however, improved blood circulation in the brain. In humans, aerobic exercise can also improve cognitive performance.
One says that specific “aging genes” are switched on at a certain time in life. Another points to the accumulation of genetic mutations or other types of DNA damage. Other researchers implicate hormonal influences or suggest that an immune system gone awry plays a central role in aging.
Finally, many researchers advance a theory of brain aging that emphasizes the inexorable accumulation of oxidative damage caused by free radicals, cell byproducts that destroy fats and proteins vital to normal cell function.
As a logical consequence of this uncertainty about what causes normal brain decline, we are equally uncertain about what sustains healthy brain function as we grow older. Increasingly, both physical and mental exercise is viewed as an effective means of slowing the effects of brain aging, perhaps by altering the levels of certain neurotropic factors that are beneficial to brain functioning.
Although much has been learned about the aging brain, many questions remain.
For instance,
does the production of proteins decline with age in all brain neurons?
In a given neuron, does atrophy lead to a higher likelihood of death?
How does aging affect gene expression in the brain — the organ with the greatest number of active genes?
Do hormonal changes at menopause contribute to gender differences in brain aging?
Neuroscientists, too, speculate that certain genes may be linked to events leading to cell death in the nervous system. By understanding the biology of the proteins produced by genes, scientists hope to be able to influence the survival of neurons and develop ways to improve their functioning. Our understanding of brain function has evolved over many years of research.
Much of this research:
-
with animals
-
imaging techniques
Thus, with age, the brain is affected in the following ways:
-
Speed declines
-
Vocab improves
-
Increasaed crystallized, decreased fluid
-
Expansion of roles depends on overall resiliency
-
Cortex neurons stay, but their connections change
-
Rodents & primates decline in spatial tasks (navigate to food)
-
Challenging environment neurons form longer dend's in cereb cort
-
however; changes are sluggish and not as large
-
-
Acrobatic preformance in rodents increased more synapses per brain cell than physical exercise and sedentary lifestyle
Theories of individual differences in aging:
-
Genetic mutations
-
Hormonal influences
-
Aging genes "turn on"
-
Immune system gone awry
-
Other DNA damage (ex. radiation, oxidative stress by free radicals)
Plausible influences on Aging:
-
physical and mental exercise (neurotropic factors )
-
does the production of proteins decline with age in all brain neurons?
-
does atrophy lead to a higher likelihood of death?
-
How does aging affect gene expression in the brain?
-
certain genes may be linked to events leading to cell death
SLEEP
We spend nearly one-third of our lives asleep AND YET WE DONT KNOW WHY WE DO IT
an endogenous rhythm that gradually becomes locked to the day-night cycle through the first years of life
Sleep is crucial for:
-
memory
-
coordination
-
concentration
-
emotional health
It is what is called a circadian rhythm - so called because ‘circa’ is Latin for around, and ‘dies’ for day.
-
babies sleep for short periods during both the day and the night
-
up to 18 hours per day
-
spend more time in deep SWS
-
-
young children often take a nap after lunch
-
less time asleep
-
less time in deep SWS
-
-
adults generally sleep only at night
-
six to seven hours per night
-
complain of inevitable early waking
-
spend very little time in slow wave sleep
-
Without enough sleep, people have trouble focusing and responding quickly when they need to, such as when they’re behind the wheel of a car.
In fact, sleep loss can have as great an effect on performance as drinking alcohol.
And growing evidence suggests that a lack of sleep increases the risk of a variety of health problems:
-
diabetes
-
stroke
-
obesity
-
infections
-
depression
-
heart attacks
-
high blood pressure
-
cardiovascular disease
Although much research has been done on sleep, it remains one of the great mysteries of modern neuroscience.
Gained understanding some of the brain circuitry that controls wake-sleep states.
Scientists now recognize:
-
sleep consists of several different stages
-
choreography of sleep involves the interplay of these stages
-
depends on a complex switching mechanism between sleepwake states.
-
-
-
Sleep stages are accompanied by daily rhythms in:
-
hormones
-
body temperature
-
+ other functions
-
Why do we sleep?
-
Theory 1: sleep is just a convenient way for animals to be kept immobile and so out of danger.
-
Theory 2: REM sleep and certain phases of SWS enable the brain to recover.
-
which occurs during the first 4 hours of the night.
-
helps to reset things in the brain and that a good time to do this necessary task is when the brain is not processing sensory information, or being vigilant and attentive, or having to control our actions.
-
-
Research: sleep is the time when we consolidate what we have learned the day before - an essential process in memory.
Sleep disorders are among the nation’s most common health problems, affecting up to 70 million people, most of whom are undiagnosed and untreated.
These disorders are one of the least recognized sources of disease, disability, and even death, costing an estimated $15.9 billion annually.
Brain Activity During Sleep
These stages were discovered in the 1950s through experiments using electroencephalography (EEG) to examine human brain waves. Researchers also measured movements of the eyes and the limbs. In EEG, electrodes placed around the head record electrical activity of the human brain in response to a variety of stimuli and activities — even sleep.
Sleep Studies Show General patterns of Sleep Mechanisms
Before; When we are Awake
-
Our brains show low-amplitude electrical activity.
The First Hour (slow-wave sleep) (SWS)
-
EEG becomes flatter at first
-
brain progresses through a series of stages during which brain waves slow down
-
slow wave sleep is accompanied by relaxation of the muscles and the eyes
-
Heart rate, blood pressure, and body temperature all fall.
-
If awakened during this time, most people recall only fragmented thoughts, not active dreams
-
gradually, increases in amplitude and decreases in frequency as we move through a series of discrete stages of sleep.
-
neurons become unresponsive to inputs, gradually becoming synchronised with each other
The Next Half Hour
-
brain activity alters drastically, from deep slow wave sleep to REM, characterized by neocortical EEG waves similar to those observed during waking.
-
Paradoxically, the fast, waking-like EEG activity is accompanied by atonia, or paralysis of the body’s muscles.
-
Only the muscles that allow breathing and control eye movements remain active.
-
During REM sleep, active dreaming takes place.
-
Heart rate, blood pressure, and body temperature become much more variable.
-
The first REM period usually lasts 10 to 15 minutes.
-
Men often have erections during this stage.
Next REM bits
-
EEG becomes like the waking state again
-
eyes jerk back and forth beneath our closed eyelids
-
more likely to dream
-
awoken during REM= `I was dreaming you fucker!`
-
about 4 to 6 short episodes of REM sleep each night
-
Babies have a bit more REM sleep
-
animals show REM sleep
cycle back and forth between these different stages of sleep
cycles of slow wave and REM sleep alternate, with the slow wave sleep becoming less deep and the REM periods more prolonged until waking occurs.
Over the course of a lifetime, the pattern of sleep cycles changes. Infants sleep up to 18 hours per day, and they spend much more time in deep slow wave sleep.
As children mature, they spend less time asleep and less time in deep slow wave sleep.
Older adults may sleep only six to seven hours per night.
What’s more, adults often complain of early waking that they cannot avoid and spend very little time in slow wave sleep.
How is sleep regulated?
which brain systems are activated?
-
maintained by several brain systems
-
each regulating different aspects of this state.
Major areas in the brain involved in the regulation of sleep
-
Upper brainstem
-
nerve cells using the following NTs connect with the forebrain:
-
ACh
-
Npa
-
5HT
-
GM
-
-
The brainstem arousal center supplies the acetylcholine for the thalamus and brainstem, and the forebrain center supplies the cerebral cortex.
-
Activation in these centers alone can create REM sleep
-
-
Hypothalamus
-
Nerve cells containing
-
orexin important in wakefulness (their loss causes narcolepsy)
-
-
histamine
-
activation of the thalamus and the basal forebrain by acetylcholine is particularly important in maintaining activity in the cerebral cortex and consciousness.
-
this level of alertness is reflected in an activated, low-voltage EEG.
-
-
Activation of other neurons that make the neurotransmitters norepinephrine, serotonin, and histamine is needed for waking.
-
The brainstem arousal center supplies the acetylcholine for the thalamus and brainstem, and the forebrain center supplies the cerebral cortex. Activation in these centers alone can create rapid eye movement sleep. Activation of other neurons that make the neurotransmitters norepinephrine, serotonin, and histamine is needed for waking.
How Sleep is Regulated
-
During non-REM sleep, the arousing systems of the upper Bst and Hus become much less active, and the transmission of information from the senses through the thalamus is curtailed.
-
Consciousness lessens, and wakefulness gives way to the slow wave pattern typical of the first stage of sleep.
-
During this state, there is active suppression of arousal systems by a group of nerve cells in the Hus, called the ventrolateral preoptic (VLPO) nucleus.
-
The cells in the VLPO contain the inhibitory Nts galanin and GABA. Damage to the VLPO nucleus produces irreversible insomnia.
Damage to the VLPO nucleus produces irreversible insomnia.
What is REM sleep?
The state of REM sleep is characterized by an internally activated brain and an activated EEG — but with external input suppressed. Internal activation during REM comes from a cyclically active REM sleep generator made up of neurons in the brainstem. Signals from these neurons cause the forebrain to become excited and lead to the rapid eye movements and muscle suppression — hallmark signs of this state.
In the absence of external input, forebrain excitation from internal sources is the driving force behind the vivid dreams experienced during REM sleep.
Interestingly, our motor cortex nerve cells fire as rapidly during REM sleep as they do during waking movement, a fact that explains why movement can coincide with dreams.
The periodic recurrence of REM sleep about every 90 minutes during sleep is thought to be caused by the on-off switching of REM-generating neurons, which produce acetylcholine and glutamate, and REM-suppressive neurons, which produce norepinephrine, serotonin, and GABA.
What Causes Sleepiness?
There are two main determining factors:
-
the circadian system (time of day or night)
-
retina->SCN->SPVoN->DMNoHus->>VLPON and orexin neurons in the lateral hypothalamus
-
-
how long we have been awake. (homeostatic system)
-
increase in sleep-inducing factor(s)
-
adenosine (inhibitory)
-
-
What is the circadian rhythm?
-
regulated by the suprachiasmatic nucleus
-
small group of cells in the Hus (master clock) just above the optic chiasm called the suprachiasmatic nucleus
-
the neurons here have many synapses between their dendries to synchronise the patterned firing
-
it ticks a bit slower than a day (is 25 hrs) kept in register by eye telling it when it is day or night
-
They express clock proteins, which go through a biochemical cycle of about 24 hours, setting the pace for daily cycles of activity, sleep, hormone release, and other bodily functions.
-
Researchers first identified these proteins and determined their roles in sleep via fruit fly Drosophila melanogaster.
Suprachiasmatic nucleus
-
receives input directly from the retina
-
clock can be reset by light so that it remains linked to the outside world’s day-night cycle
-
provides signals to an adjacent brain area called the subparaventricular nucleus
-
in turn contacts the dorsomedial nucleus of the hypothalamus
-
dorsomedial nucleus then contacts the ventrolateral preoptic nucleus and the orexin neurons in the lateral hypothalamus
-
It is these neurons that directly regulate sleep and arousal.
Orexin
-
provides an excitatory signal to the arousal system, particularly to the norepinephrine neurons.
-
recent work using selective stimulation of orexin neurons by artificially inserted receptors sensitive to fiberoptic light pulses produces arousal.
-
This arousal is mediated by orexin activation of norepinephrine neurons in the locus coeruleus.
-
Orexin activation plays a critical role in preventing abnormal transitions into REM sleep during the day, as occurs in narcolepsy.
Mice Experimentation
1. Gene for the NT orexin was experimentally removed, became narcoleptic.
Narcoleptic humans: the orexin levels in the brain and spinal fluid are abnormally low.
2. Around every 25 days, Mice switch their sleep cycles forward by 8 hours. Mice genesgenes expressed in the suprachiasmatic nucleus causing jetleg (VIPR2) were knocked out and, as expected, the mice didn`t experience jetlag, while normal mice took time to readjust. Day-night activity patterns were normal only until day 25. These kinds of studies should help us learn about the molecular mechanisms by which light entrains circadian pacemaker genes.
How do rhythms work?
-
EEG reveals a brainstem activating system involving various NM transmitters, ex. adenosine, in a kind of molecular chain reaction that takes us through the various sleep stages.
-
Synchronisation mechanisms enable networks to pass from one sleep state to another.
-
A big leap forward has come from neurogenetics.
-
Various genes have been identified that are the molecular components of rhythmical pacemakers.
-
Ex. Drosophila: two genes - per and tim - produce proteins that interact together to regulate their own synthesis.
-
mRNA and protein synthesis begins early in the day, the proteins accumulate, link up together and this linkage then stops their own synthesis.
-
Daylight helps to degrade the proteins whose level eventually drops to a point where the genes that make PER and TIM protein get going again.
-
This cycle goes round and round, and will even carry on if the neurons are kept alive in a dish.
-
-
The clock in mammals such as ourselves operates in a remarkably similar way to the one in flies.
-
As circadian rhythms are very old in evolutionary terms, it is perhaps no surprise that the same type
Homeostatic system
-
The second system regulating sleepiness is the homeostatic system, which responds to progressively longer wake periods by increasing the urge to sleep.
-
The subjective sense of the increasing need to sleep coinciding with increasing wakefulness suggests that there might be a brain physiological parallel; that is, the longer a person is awake, the greater the likelihood of an increase in sleep-inducing factor(s).
-
Evidence now suggests that one important sleep factor is the inhibitory neurochemical adenosine.
Adenosine
-
With prolonged wakefulness, increasing levels of adenosine are evident in the brain, initially in the basal forebrain and then throughout the cortex.
-
The increased levels of adenosine serve the purpose of slowing down cellular activity and diminishing arousal.
-
Adenosine levels then decrease during sleep.
-
These studies of adenosine prompted examination of the ATP, the cellular energy source that powers nerve cells in the brain.
-
Brain adenosine may be produced by ATP breakdown in the course of the high brain activity that takes place during wakefulness.
-
Since nerve cell activity decreases and adenosine levels decline in nonREM sleep, the logical assumption is that ATP increases during sleep.
-
Indeed, studies in animals found that brain ATP levels soared during the initial hours of non-REM sleep.
Because ATP is needed to produce adenosine, which is essential for wakefulness, it makes sense that ATP is produced during sleep. This finding also supports the commonly held notion that sleep is necessary for providing restorative energy.
Indeed, recent work using selective stimulation of orexin neurons by artificially inserted receptors sensitive to fiberoptic light pulses — a process referred to as optogenetic stimulation — produces arousal.
Sleep disorders are among the nation’s most common health problems, affecting up to 70 million people, most of whom are undiagnosed and untreated.
These disorders are one of the least recognized sources of disease, disability, and even death, costing an estimated $15.9 billion annually.
Sleep Disorders
The most common SD, and the one most people are familiar with, is insomnia.
-
difficulty falling asleep initially
-
others fall asleep and then awaken partway through the night and cannot fall asleep again.
short-acting sedatives and sedating antidepressant drugs help, but none completly; they suppress deeper stages of slow wave sleep, not effective in helping people stay asleep.
Many of the most common disorders, listed below, disrupt sleep and result in inadequate amounts of sleep, particularly of the deeper stages.
-
Excessive daytime sleepiness, which has many causes.
-
Obstructive sleep apnea
-
occurs as sleep deepens and the airway muscles in the throat relax to the point of collapse (closing the airway)
-
difficulty breathing and wakes up without entering the deeper stages of slow wave sleep
-
condition can cause high blood pressure and may increase the risk of heart attack
-
Increased daytime sleepiness that results from sleep apnea can lead to an increased riskof daytime accidents, esp. automobile accidents.
-
Treatment
-
reduce airway collapse during sleep
-
losing weight, avoiding alcohol and sedating drugs prior to sleep
-
avoiding sleeping on one’s back can sometimes help
-
most people require devices that induce continuous positive airway pressure
-
small mask that fits over the nose to provide an airstream under pressure during sleep
-
-
In some cases, surgery is needed to correct the airway anatomy.
-
-
-
Periodic limb movements and REM behavior disorder
-
intermittent jerks of the legs or arms
-
occur as the individual enters slow wave sleep
-
can cause arousal from sleep
-
Similar: REM behavior disorder
-
muscles fail to become paralyzed during REM sleep
-
act out dreams
-
-
more common in people with Parkinson’s disease, and both can be treated with drugs for Parkinson’s or with a benzodiazepine called clonazepam.Narcolepsy
-
-
Narcolepsy
-
one case per 3,000 people (uncommon)
-
switching mechanisms controlling the transitions into sleep, particularly REM sleep, do not work properly
-
due to the loss of nerve cells in the lateral hypothalamus that contain the neurotransmitter orexin (also known as hypocretin)
-
tend to enter REM sleep very quickly as well and may even enter a dreaming state while still partially awake, a condition known as hypnagogic hallucination.
-
attacks during which they lose muscle tone — a state similar to what occurs during REM sleep but instead happens while they are awake.
-
These attacks of paralysis, known as cataplexy, can be triggered by emotional experiences, even by hearing a funny joke.
-
studies study processes that control these transitions between waking, slow wave sleep, and REM sleep states.
-
-
Randy Gardner
-
264 hours without sleep
-
Guinness book of Records
-
longest period ever recorded
-
carefully controlled experiment
-
supervised by doctors in the American Navy
-
main difficulties:
-
with speech
-
inability to concentrate
-
lapses of memory
-
hallucinatory daydreaming
-
-
body remained in excellent physical condition
-
never became psychotic or lost contact with reality
-
slept fifteen hours the first night and short extra periods on succeeding nights.
-
This and others convinced researchers that primarily the brain and not body that really gains from sleep.
-
Similar carefully controlled animal experiments.
In summary
Sleep; its good because
-
memory
-
coordination
-
concentration
-
emotional health
and its lack can cause problems such as
-
diabetes
-
stroke
-
obesity
-
infections
-
depression
-
heart attacks
-
high blood pressure
-
cardiovascular disease
-
even death, if you fall asleep behind the wheel
Over the course of a lifetime:
-
sleep less
-
less time in deep SWS
-
wake up more
NTs involved in Sleep:
-
The brainstem arousal center supplies the acetylcholine for the Tus and BSt
-
forebrain center supplies the cerebral cortex.
-
-
Activation in these centers alone can create REM sleep.
-
Activation of other neurons that make the NTs Npe, 5HT, and histamine is needed for waking.
Sleep is patterned; It is controlled by
-
Circadian rhythm
-
small group of cells in the Hus just above the optic chiasm called the suprachiasmatic nucleus
-
the neurons here have many synapses between their dendries to synchronise the patterned firing
-
it ticks a bit slower than a day (is 25 hrs) kept in register by eye telling it when it is day or night
-
sleep experiments living in deep caves for long time adopt patterns of activity that free-run to a sleepwaking cycle of about 25 hours.
-
-
What happens during sleep
-
awake, our brains show low-amplitude electrical activity.
-
fall asleep: EEG becomes flatter at first
-
gradually, increases in amplitude and decreases in frequency as we move through a series of discrete stages of sleep.
-
These stages are called slow-wave sleep (SWS).
-
reasons for these changes????
-
as neurons in the brain become unresponsive to their normal inputs, they gradually become synchronised with each other.
-
lose muscle tone as the neurons controlling skeletal muscle movements are actively inhibited but the ones controlling respiration and heart rate carry on working normally
-
-
-
cycle back and forth between these different stages of sleep
-
REM stage of sleep
-
EEG becomes like the waking state again
-
eyes jerk back and forth beneath our closed eyelids
-
more likely to dream
-
awoken during REM= `I was dreaming you fucker!`
-
about 4 to 6 short episodes of REM sleep each night
-
Babies have a bit more REM sleep
-
animals show REM sleep
-
Around every 25 days, Mice switch their sleep cycles forward by 8 hours. Mice genesgenes expressed in the suprachiasmatic nucleus causing jetleg (VIPR2) were knocked out and, as expected, the mice didn`t experience jetlag, while normal mice took time to readjust. Day-night activity patterns were normal only until day 25. These kinds of studies should help us learn about the molecular mechanisms by which light entrains circadian pacemaker genes.
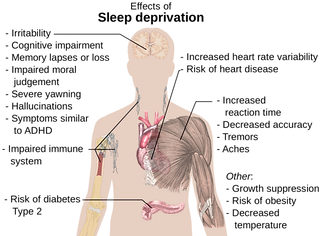
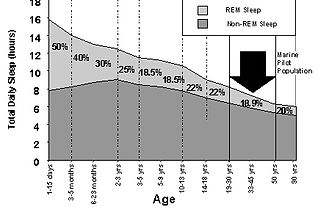
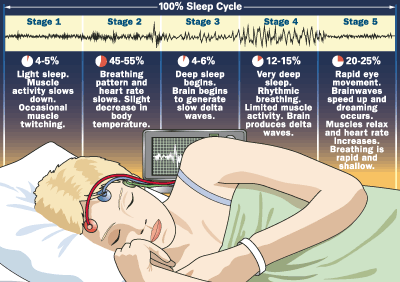
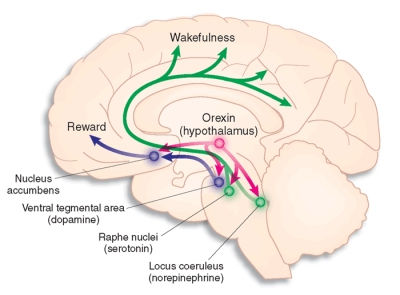
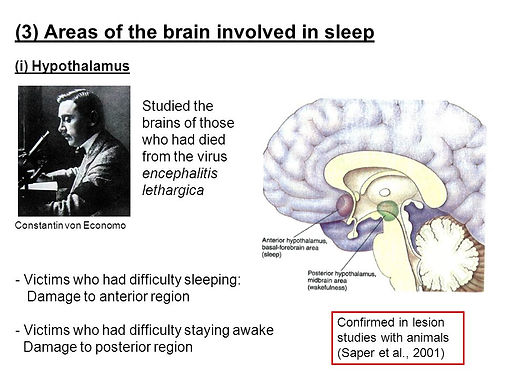
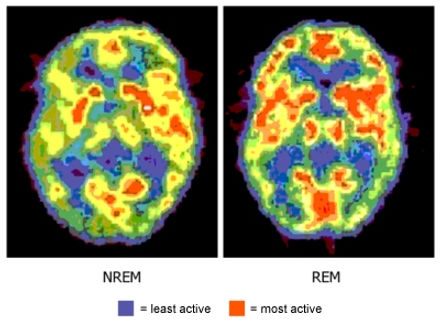
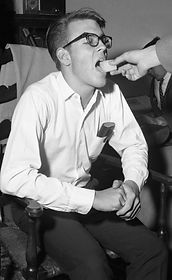
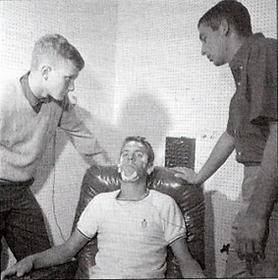

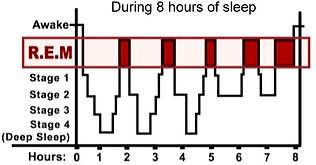

